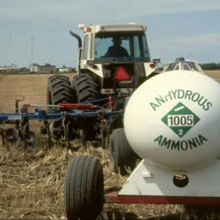
Higher N pricesand environmental concerns make field application choices more important.
Risks associated with fall nitrogen (N) applications fit into four categories: logistical, agronomic, environmental and economic, the last of which currently ties very closely to the others and will be discussed in conjunction with them.
Logistical risks
The primary reason growers apply N in the fall is the flexibility it allows in N management. Fall is typically drier than spring and offers more days suitable for field work. Farmers considering shifting their N applications from fall to spring must carefully evaluate the additional workload spring requires. Many regular spring field operations must be performed within a short time period. If farmers plant corn too late, they can incur significant yield and economic losses. If the spring rush causes equipment to run over ground that’s too wet, soils can become compacted, compounding yield and economic losses that can last several years.
Agronomic risks
Fall applications of N are, at best, equal to in-season applications of N in terms of effectiveness. They are seldom superior. They carry a higher risk of lower production arising from N loss between application and crop need. To minimize agronomic risk, university extension publications and industry professionals recommend applying it when daily soil temperatures are cooler and typically sustain below 50⁰F (Snyder et al., 2000). Nitrifying organisms that convert ammonium (NH+) N₄ to nitrate (NO₃-) are, for the most part, inactive until temperatures rise above 40⁰F (Schmidt, 1982). At temperatures above this level, nitrification rates start out slowly but increase rapidly and can vary by soil (Sabey et al., 1956). So by applying N in the fall when soils are cooler, better chances exist that enough N will still be present for corn production the next year.
Environmental risks
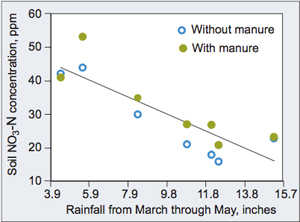
Variations within 0 to 12 inches of early-June soil NO₃- levels across different years, and of March-through-May rainfall totals (Balkcom et al., 2003).
Compared with in-season applications, applying N in the fall increases the chances that leaching will cause some portion of the N to be lost prior to crop uptake. In a multiple-year Iowa study (Balkcom et al., 2003), soil NO₃-N concentrations in early June were well related to the amount of total rainfall received in March, April and May ( Figure 1). As rainfall during this period increased, less NO₃-N was present in the upper 12 inches of soil, indicating losses had occurred. This study illustrates that probabilities of N loss can be highly related to weather. Consequently, farmers can reduce the risk of N loss through leaching by applying N closer to the time of crop need.
If in-season N applications are rushed and soils become compacted, risks of N loss through denitrification (gaseous loss as N2O, NO or N2) may increase. The emission of nitrous oxide (N2O), a greenhouse gas, has been shown to be higher under compacted soil conditions. A study in Germany demonstrated that inter-rows compacted by wheel traffic emitted over six times as much N2O as non-compacted inter-rows and accounted for 68 percent of the total N2O losses from the field (Ruser et al., 1998).
Besides losing gaseous N, compacted soils can also reduce total N uptake by the crop (Figure 2). Lower uptake leads to yield losses and increases the quantity of N left in the soil after the cropping season. Depending on conditions, this unused N may be subject to losses.
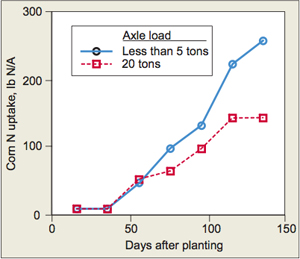
Effects of wheel-traffic-induced soil compaction on N uptake (Wolkowski, 1990).
Appropriate N sources for fall
Nitrate sources.
Sources of N containing NO₃- are not recommended for fall application for spring-planted corn. Soils don’t hold in nitrate, so it moves with water through the soil profile. Such N sources therefore run a high risk of NO₃- leaching and runoff losses when crops are not present or actively growing for uptake. Common fertilizer products with such restrictions include urea ammonium nitrate (UAN) and ammonium nitrate.
Ammonium sources.
Ammonium, however, is held by the soil and does not readily leach. Ammonium sources are therefore suitable for fall applications. Commonly applied forms of N in this category include ammonium sulfate, diammonium phosphate, and monoammonium phosphate.
Ammonia sources.
Many consider anhydrous ammonia (NH₃) to be the best source to apply in the fall because it can delay nitrification. An early laboratory study (Eno and Blue, 1954) showed that this effect depends on soil type and soil pH, among other factors ( Table 1). On a slightly basic Arredondo loamy fine sand, NH₃ nitrified more slowly than ammonium sulfate. However, the reverse was true on a slightly acidic Lakeland fine sand. The temporary pH increases following NH₃ applications may be favorable for nitrifying organisms at lower pH levels, but unfavorable at higher soil pH levels.
Urea sources.
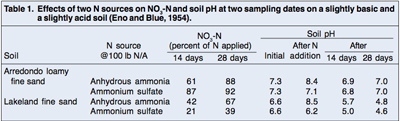
Use of urea is acceptable for fall applications in most climates, if it’s incorporated soon after application on soils with relatively low leaching and denitrification loss potentials. Once incorporated (by tillage or rainfall) and in the presence of adequate moisture, urea readily converts to NH + because of the action of soils. Movement of urea-N in soils is akin to NO₃- movement, so fall applications in sandy soils with a high leaching potential is generally not recommended.
Appropriate inhibitors for fall-applied N
Nitrification inhibitors.
To limit denitrification losses, research recommends nitrification inhibitors in higher-rainfall areas of the Corn Belt with poorly drained soils and soils with higher moisture levels near the surface. Throughout the region, fall applications with a nitrification inhibitor have tended to be less effective in reducing the risk of losses at the same rate of N applied in the spring (Randall et al., 2003).
New evidence indicates that pH may also play an important role in determining effectiveness of nitrification inhibitors. Figure 3 shows that in a year with warmer December and February temperatures, effectiveness of a nitrification inhibitor was greater than in a year with colder December, February and March temperatures. In the warmer year, higher soil pH levels probably led to a more rapid recovery of nitrifying organisms from the inhibitor, resulting in a greater amount of nitrification at the time of measurement.

The influence of pH on the percentage of fall-applied N that had been nitrified by the following spring, with and without a nitrification inhibitor. Sub-figure “a” shows years with above-average spring precipitation, and sub-figure “b” shows years with below-average spring precipitation (Kveryga et al., 2004).
Urease inhibitors.
For urea forms of N, a urease inhibitor may provide benefits when the incorporation of 0.3 to 0.5 inches of rain doesn’t occur within 2 to 3 days after application (Fox and Piekielek, 1993).
References
Balkcom, K.S., A.M. Blackmer, D.J. Hansen, T.F. Morris, and A.P. Mallarino. 2003. Testing soils and cornstalks to evaluate nitrogen management on the watershed scale. J. Environ. Qual. 32:1015-1024.
Eno, C.F. and W.G. Blue. 1954. The effect of anhydrous ammonia on nitrification and the microbiological population in sandy soils. Soil Sci. Soc. Am. Proc. 18:178-181.
Fox, R.H. and W.P. Piekielek. 1993. Management and urease inhibitor effects on nitrogen use efficiency in no-till corn. J. Prod. Agric. 6:195-200.
Kveryga, P.M., A.M. Blackmer, J.W. Ellsworth, and R. Isla. 2004. Soil pH effects on nitrification of fall-applied anhydrous ammonia. Soil Sci. Soc. Am. J. 68:545-551.
Randall, G.W., J.A. Vetsch, and J.R. Huffman. 2003. Corn production on a subsurface-drained mollisol as affected by time of nitrogen application and nitrapyrin. Agron. J. 95:1213-1219.
Ruser, R., H. Flessa, R. Schilling, H. Steindl, and F. Beese. 1998. Soil compaction and fertilization effects on nitrous oxide and methane fluxes in potato fields. Soil Sci. Soc. Am. J. 62:1587-1595.
Sabey, B.R., W.V. Bartholomew, R. Shaw, and J. Pesek. 1956. Influence of temperature on nitrification in soils. Soil Sci. Soc. Am. Proc. 20:357-360.
Schmidt, E.L. 1982. Nitrification in soil. p. 253-288. InF.J. Stevenson (ed.) Nitrogen in agricultural soils. Agron. Monogr. 22. ASA/CSSA/SSSA, Madison, Wis.
Snyder, C.S., G.W. Randall, R.E. Lamond, and R.G. Hoeft. 2000. Fall nitrogen management for agronomic response and environmental protection [online]. Available at >http://www.ppi-ppic.org/falln<.
Wolkowski, R.P. 1990. Relationship between wheel-traffic-induced soil compaction, nutrient availability, and crop growth: A review. J. Prod. Agric. 3:460-469.